Electricity Batteries
Storing electricity is one of the key components for a renewable energy future. There are many mechanisms to store electrical energy:
-
Chemical batteries
-
Gravity batteries
-
Mechanical batteries
Each of these categories of batteries have their advantages and disadvantages. The key factors to consider when designing an electricity storage solution are:
-
Duration – how long do you need the battery to provide power
-
Lifespan – how many charge / discharge cycles will the technology support
-
Operational mode – what business purpose is the battery to address
-
Round trip efficiency – how much electricity (kWh) to you get back for the energy input
-
Maintenance costs
-
Physical size
-
Lifecycle cost – including capital cost, maintenance cost and replacement costs
Operational Modes
Electricity batteries are normally considered to address a number of business challenges
-
Backup power to cover operational needs during a grid failure
-
Electricity storage for renewable / intermittent generation
-
Grid rate arbitrage
-
Peak demand management
-
Frequency control
For backup power, the battery design needs to consider the power (kW) required to support the critical systems as well as the energy duration (kWh). Both these factors affect the cost of the battery solution. However, different types of batteries are more cost effective for short term power and others are more cost effective for longer duration energy storage. Chemical batteries such as Lithium Ion are cost effective for short duration systems (1 to 4 hours), where salt water batteries are much more cost effective for long duration storage.
Electricity storage to capture excess electricity from intermittent generation (such as solar PV) needs to consider the maximum power from the intermittent source and the total energy required for storage before it is discharged. For example, a 100kW solar PV array at equatorial latitudes where electricity is created 10 hours per day may want storage for 14 hours (for 24 hour coverage). In this case, chemical batteries become very expensive and a more cost effective solution can be found with salt water batteries (see case study).
Grid rate arbitrage is relevant for those regions where the electricity rates vary hour by hour. For example, the electricity rates in Alberta Canada can vary from $0/MWh to $999/MWh depending on the current demand and generation sources. Batteries can help facilities charge the battery when the grid rates are low and then discharge when the grid rates are high. In this case, a deep analysis on the frequency and duration of the grid rate spikes is required to design the optimal battery solution (power and energy).
​
Peak demand management can benefit from a battery deployment. In most cases, the electricity utility will include a charge for the peak power demand at the facility – and in some instances this can be a significant component of the electricity costs. Batteries can capture electricity when the building demand is low, and then discharge when the building demand exceeds a threshold. The objective is to level out the buildings grid electricity consumption. In this case, an analysis of the facilities electricity demand is required. How large is the peak demand (how much power do we need to provide)? How long are these peak demand periods (how much energy do we need to store)? The longer the peak demand periods, the more energy is required in the battery. A short term peak (less than an hour) can be addressed with standard Lithium Ion batteries.
The ability of a Battery Energy Storage System (BESS) to produce active power output in response to a shift in the electrical grid's frequency is known as its frequency responsiveness. A mismatch between the system's power supply and demand is shown when the grid's frequency deviates from its nominal frequency. Restoring the supply and demand balance as soon as possible is crucial to preserving the grid's stability and dependability. By modifying their active power output in response to a frequency variation, BESS can provide frequency responsiveness. A droop characteristic, which describes the connection between the frequency deviation and the active power output, can be used to manage the BESS. The amount of active power output is exactly proportional to the degree of frequency variation, exhibiting a proportional connection known as the droop characteristic. The BESS will raise its active power output in proportion to the degree of frequency deviation when the grid's frequency drops (underfrequency).
Chemical Batteries
The most popular chemical battery is a Lithium Ion battery. These are used in mobile applications as they have higher energy density than other technologies. As such they are used in phones and electric vehicles. However, Lithium Ion batteries have a limited number of charge / discharge cycles before they reach the end of useful life. Lithium Ion batteries are ideal for short term electricity storage (less than 12 hours for a phone, 1 hour for a UPS for computer backup power, less than 4 hours for a building backup system).
Lead acid batteries can be charged/discharged more often but are large and heavy and not well suited for mobile applications.
Salt water batteries address the medium to long term electricity storage market (more than 4 hours). Salt water batteries have the advantage that extending the energy (kWh) is relatively inexpensive. However, they are very large (sea container size) and are better suited for applications where space is not a concern.
Gravity Batteries
A gravity battery works by using excess energy (usually from sustainable sources) to raise a mass to generate gravitational potential energy, which is then lowered to convert potential energy into electricity through an electric generator. There are a number of electricity storage systems that leverage gravity including heavy lift and pumped hydro.
An example of a heavy lift battery utilizes abandoned vertical mine shafts. In this case, a heavy load is raised to the surface to store potential energy. When electricity is required, the heavy load is lowered which turns a generator. An example of this is offered by Energy Vault.
Pumped hydro leverages the difference in altitude of the local terrain. Water is pumped from a lower altitude to a higher altitude reservoir such as a lake or dam. When electricity is required, the water is released which turns a generator in the same manner as typical hydro-electric plants. The round trip efficiency of these batteries is typically 70 to 80%. Pumped storage hydropower costs $165/kWh to operate, with a levelized cost of storage (LCOS), of $0.17/kWh. These batteries have the advantage that they have no limitations in charge/discharge cycles. However, these batteries are only realistic in regions that have hydro-electric potential – regions with beneficial terrain.
Mechanical Batteries
Flywheel energy storage (FES) works by accelerating a rotor (flywheel) to a very high speed and maintaining the energy in the system as rotational energy. When energy is extracted from the system, the flywheel's rotational speed is reduced as a consequence of the principle of conservation of energy; adding energy to the system correspondingly results in an increase in the speed of the flywheel. The energy efficiency of flywheels, also known as round-trip efficiency, can be as high as 90%. Typical capacities range from 3 kWh to 133 kWh. Rapid charging of a system occurs in less than 15 minutes.
Flywheel energy storage systems have improved their efficiencies by moving from mechanical bearings (which created maintenance events) to magnetic bearings. They have also encased the flywheel in a vacuum to reduce rotational drag.
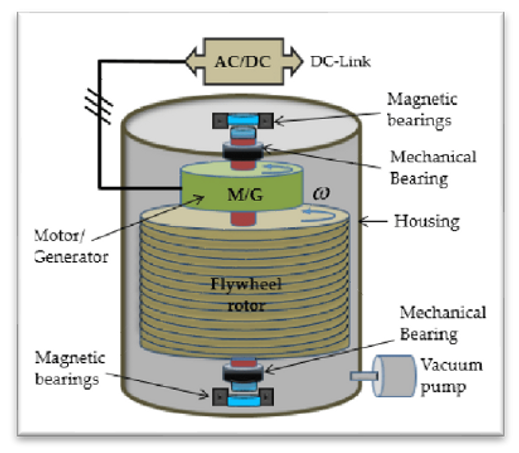